Abstracts
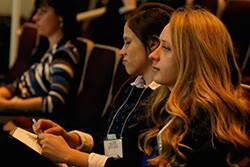
Applicants for UCUR prepare an abstract summarizing the nature of the endeavor and its results and highlighting interesting outcomes. Some institutions use the abstract to judge whether or not to accept the student to represent the school at UCUR. Please write the abstract for a non-expert but educated person.